Introduction
Necroptosis, a form of regulated cell death, has gained significant attention recently due to its involvement in various physiological and pathological processes. Necroptosis is a specialised form of programmed cell death that is tightly controlled by various signalling pathways, mediators, and immune responses. Interferon-γ (IFN-γ) and Toll-like receptor 4 (TLR4) signalling pathways have been identified as critical regulators of necroptosis in macrophages. Understanding the underlying molecular mechanisms that govern necroptosis is essential for unravelling its role in immune responses and disease pathogenesis.
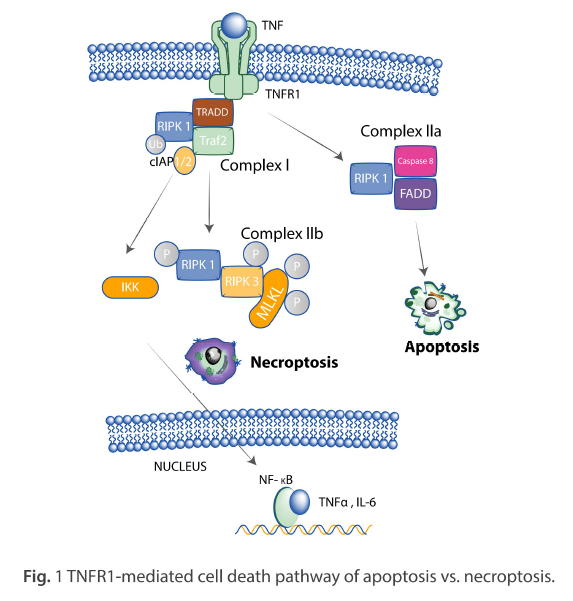
This white paper introduces the recent findings in molecular mechanisms that govern necroptosis as they relate to receptor-interacting protein kinases (RIPK) and provides examples of ProSci’s rabbit anti-RIPK3 antibody (ProSci Cat #: 2283) when used to analyse the expression of RIPK3 in various cell lines by Western Blot.
ProSci LLC’s portfolio brings more than 25 years of antibody expertise in developing high-quality single-domain Variable Heavy domain of Heavy chain (VHH), recombinant, monoclonal, and polyclonal antibodies. In addition, ProSci’s custom antibody services provide the flexibility to tailor antibody development and production for almost any need.
“RIPK3 and MLKL: Essential Players in IFN-γ Induced PS Exposure and Necroptosis”
The paper published in 2019 by Chen et al. titled “Interferon induces the cell surface exposure of phosphatidylserine (PS) by activating the protein MLKL in the absence of caspase-8 activity” presents research findings on the role of RIPK3 in IFN-γ-induced PS exposure in mouse embryonic fibroblasts (MEFs). The authors demonstrated that RIPK3 is necessary for PS exposure in MEFs. At the same time, RIPK3-activated oligomerisation of the mixed lineage kinase domain-like protein (MLKL) protein is essential for IFN-γ induced PS exposure and the execution of necroptotic cell death in MEFs (1).
Necroptosis is a non-apoptotic, regulated form of necrosis triggered by inducers such as tumour necrosis factor (TNF) binding to their receptors (2). It requires the activity of RIPK1 and RIPK3 and is mediated by activating MLKL. (3) Interferon (IFN) is classified into two groups, type I and type II, and both types of IFN induce the expression of hundreds of specific genes (4,5).
This paper demonstrated that RIPK3 is necessary for IFN-γ-induced PS exposure and necroptosis in RIPK3-reconstituted iC8KO MEFs, immortalised caspase-8 knockout (iC8KO) mouse-derived embryonic fibroblasts with exogenously expressed RIPK3, and that MLKL is phosphorylated and oligomerised in IFN-γ treated MEFs before necroptosis. Furthermore, IFN-γ-induced PS exposure and necroptosis depend on RIPK1, RIPK3, and MLKL in various cells.
This research highlights the role of RIPK3, MLKL, and IFN-γ in inducing PS exposure and necroptosis in MEFs and provides insights into the mechanisms underlying IFN-γ-induced PS exposure and the potential functions of MLKL in this process. Aided by the use of ProSci’s rabbit anti-RIPK3 antibody, these findings could lead to the development of new therapeutic strategies for targeting necroptosis in diseases such as cancer and infection.
“Impact of RIPK1 K45A Mutation on Necroptosis, Cytokine Signaling, and Immune Response: An Altered Landscape of Inflammatory and Infection Susceptibility”
The paper published in 2016 by Shutinoski et al. titled “K45A mutation of RIPK1 results in poor necroptosis and cytokine signalling in macrophages, which impacts inflammatory responses in vivo” investigated the role of the kinase domain of RIPK1 in necroptosis and cytokine signalling in macrophages, and its consequent impact on inflammatory response in vivo. It also evaluated the effect of a K45A mutation of RIPK1 on the necroptosis of macrophages and the activation of an inflammatory response (7).
RIPK1 is essential for the induction of necroptosis and is triggered by the engagement of various Toll-like receptors (TLRs) and cytokine receptors (8,9). The phosphorylation of RIPK1 leads to the interaction of RIPK1 and RIPK3, which is necessary for necroptosis to occur (10). RIPK3 can also induce necroptosis independently of RIPK1 in certain cases (11). Furthermore, the MLKL protein is also essential for necroptosis, as its phosphorylation results in its oligomerisation and translocation to the cell membrane, disrupting cellular integrity (12). Thus, RIPK1 and MLKL proteins play vital roles in the induction of necroptosis through their respective interactions and phosphorylation.
In their study, the authors employed the RIPK1^K45A strain of mice to elucidate the crucial role of RIPK1 kinase activity in facilitating macrophage necroptosis. Through their investigations, they observed that the RIPK1^K45A mutation led to a decline in cytokine production, a reduction in the phosphorylation of RIPK1 and MLKL proteins, and an elevation in cell survival after treatments involving the combination of lipopolysaccharide (LPS) and a cell-permeable pan-caspase inhibitor (zVAD) as well as tumour necrosis factor-alpha (TNFα) and zVAD. LPS/zVAD treatment was employed to induce necroptosis in macrophages, where macrophages were exposed to LPS along with zVAD. Similarly, TNFα/zVAD treatment entailed the application of TNFα, an inflammatory cytokine, in conjunction with zVAD to induce necroptosis in macrophages.
Additionally, the researchers uncovered the regulatory influence of RIPK1 on TNFα signalling and its involvement in the transcriptional regulation of macrophage inflammatory protein-1 alpha (MIP-1α) and interleukin-1 alpha (IL-1α). Furthermore, the researchers made an intriguing observation that caspase-8 inhibitors heightened the occurrence of necroptosis in macrophages, particularly when the p38MAPK pathway was suppressed.
This study demonstrated that lysine-45 of RIPK1 is required for RIPK1 auto-phosphorylation and cell death of macrophages induced by various stimuli, including LPS and TNF-α. RIPK1K45A macrophages were resistant to cell death induced by these stimuli and showed reduced phosphorylation of STAT1 in response to LPS/ZVAD and TNF-α/ZVAD treatments. These results suggest that RIPK1K45A is involved in regulating cell death downstream of TNF-R engagement and plays a role in preventing excessive cell death in response to various stimuli.
The study showed that phosphorylation of RIPK3 was attenuated in RIPK1K45A macrophages, indicating this kinase domain’s important role in promoting macrophage necroptosis. The RIPK3 antibody was used to identify and measure the levels of RIPK3, as well as its interaction with RIPK1 and MLKL using western blot. Overall, the results suggest that the RIPK3 antibody was essential in characterising RIPK1 kinase activity and its role in regulating necroptosis, inflammatory response, and cytokine signalling in macrophages.
Conclusion
These investigations provide significant insights into the roles of RIPK3, MLKL, and IFN-γ in the initiation of phosphatidylserine presentation and necroptosis in mouse embryonic fibroblasts (MEFs). Furthermore, it underscores the crucial roles of RIPK3 and MLKL in the IFN-γ-mediated phosphatidylserine display and the necroptosis pathway.
In addition, a mutation in RIPK1, specifically RIPK1K45A, hinders the necroptosis of macrophages and the ignition of the inflammatory response. This research emphasises the role of the RIPK1 kinase domain in initiating necroptosis and cytokine signalling within macrophages and the subsequent effects on in vivo inflammatory responses.
The ProSci rabbit anti-RIPK3 antibody was employed in this investigation to examine the expression of RIPK3 across different cell lines, playing a pivotal role in delineating RIPK1 kinase activity and its regulatory functions in necroptosis, inflammatory reactions, and cytokine signalling in macrophages. The results of these investigations have the potential to facilitate additional studies into the functions of RIPK3 and MLKL in the process of phosphatidylserine exposure and necroptosis, thereby advancing our comprehension of the underlying mechanisms of cell death in the immune system that leads to the development of new therapeutic strategies for targeting necroptosis in diseases such as cancer and infection.
Visit our website to learn more about our anti-RIPK3 antibody and related antibodies needed to help with your research: caltagmedsystems.co.uk/prosci
References
- Chen J, Kuroki S, Someda M, Yonehara S. Interferon-γ induces the cell surface exposure of phosphatidylserine by activating the protein MLKL in the absence of caspase-8 activity. J Biol Chem. 2019 Aug 9;294(32):11994-12006. doi: 10.1074/jbc.RA118.007161. Epub 2019 Jun 19. PMID: 31217278; PMCID: PMC6690710.
- Dondelinger Y, Hulpiau P, Saeys Y, Bertrand MJM, Vandenabeele P. An evolutionary perspective on the necroptotic pathway. Trends Cell Biol. 2016 Oct;26(10):721-732. doi: 10.1016/j.tcb.2016.06.004. Epub 2016 Jun 28. PMID: 27368376.
- Cho YS, Challa S, Moquin D, Genga R, Ray TD, Guildford M, Chan FK. Phosphorylation-driven assembly of the RIP1-RIP3 complex regulates programmed necrosis and virus-induced inflammation. Cell. 2009 Jun 12;137(6):1112-23. doi: 10.1016/j.cell.2009.05.037. PMID: 19524513; PMCID: PMC2727676.
- Pestka S, Krause CD, Walter MR. Interferons, interferon-like cytokines, and their receptors. Immunol Rev. 2004 Dec;202:8-32. doi: 10.1111/j.0105-2896.2004.00204.x. PMID: 15546383.
- Borden EC, Sen GC, Uze G, Silverman RH, Ransohoff RM, Foster GR, Stark GR. Interferons at age 50: past, current and future impact on biomedicine. Nat Rev Drug Discov. 2007 Dec;6(12):975-90. doi: 10.1038/nrd2422. PMID: 18049472; PMCID: PMC7097588.
- Zargarian S, Shlomovitz I, Erlich Z, Hourizadeh A, Ofir-Birin Y, Croker BA, Regev-Rudzki N, Edry-Botzer L, Gerlic M. Phosphatidylserine externalization, “necroptotic bodies” release, and phagocytosis during necroptosis. PLoS Biol. 2017 Jun 26;15(6):e2002711. doi: 10.1371/journal.pbio.2002711. PMID: 28650960; PMCID: PMC5501695.
- Shutinoski B, Alturki NA, Rijal D, Bertin J, Gough PJ, Schlossmacher MG, Sad S. K45A mutation of RIPK1 results in poor necroptosis and cytokine signaling in macrophages, which impacts inflammatory responses in vivo. Cell Death Differ. 2016 Oct;23(10):1628-37. doi: 10.1038/cdd.2016.51. Epub 2016 Jun 3. PMID: 27258786; PMCID: PMC5041191.
- Kelliher MA, Grimm S, Ishida Y, Kuo F, Stanger BZ, Leder P. The death domain kinase RIP mediates the TNF-induced NF-kappaB signal. Immunity. 1998 Mar;8(3):297-303. doi: 10.1016/s1074-7613(00)80535-x. PMID: 9529147.
- Vandenabeele P, Galluzzi L, Vanden Berghe T, Kroemer G. Molecular mechanisms of necroptosis: an ordered cellular explosion. Nat Rev Mol Cell Biol. 2010 Oct;11(10):700-14. doi: 10.1038/nrm2970. Epub 2010 Sep 8. PMID: 20823910.
- Cho YS, Challa S, Moquin D, Genga R, Ray TD, Guildford M, Chan FK. Phosphorylation-driven assembly of the RIP1-RIP3 complex regulates programmed necrosis and virus-induced inflammation. Cell. 2009 Jun 12;137(6):1112-23. doi: 10.1016/j.cell.2009.05.037. PMID: 19524513; PMCID: PMC2727676.
- Upton JW, Kaiser WJ, Mocarski ES. Virus inhibition of RIP3-dependent necrosis. Cell Host Microbe. 2010 Apr 22;7(4):302-313. doi: 10.1016/j.chom.2010.03.006. PMID: 20413098; PMCID: PMC4279434.
- Sun L, Wang H, Wang Z, He S, Chen S, Liao D, Wang L, Yan J, Liu W, Lei X, Wang X. Mixed lineage kinase domain-like protein mediates necrosis signaling downstream of RIP3 kinase. Cell. 2012 Jan 20;148(1-2):213-27. doi: 10.1016/j.cell.2011.11.031. PMID: 22265413.
Originally posted on prosci-inc.com/blog/insights-into-the-molecular-mechanisms-regulating-necroptosis/
Caltag Medsystems is the distributor of ProSci products in the UK and Ireland. If you have any questions about these products, please contact us.